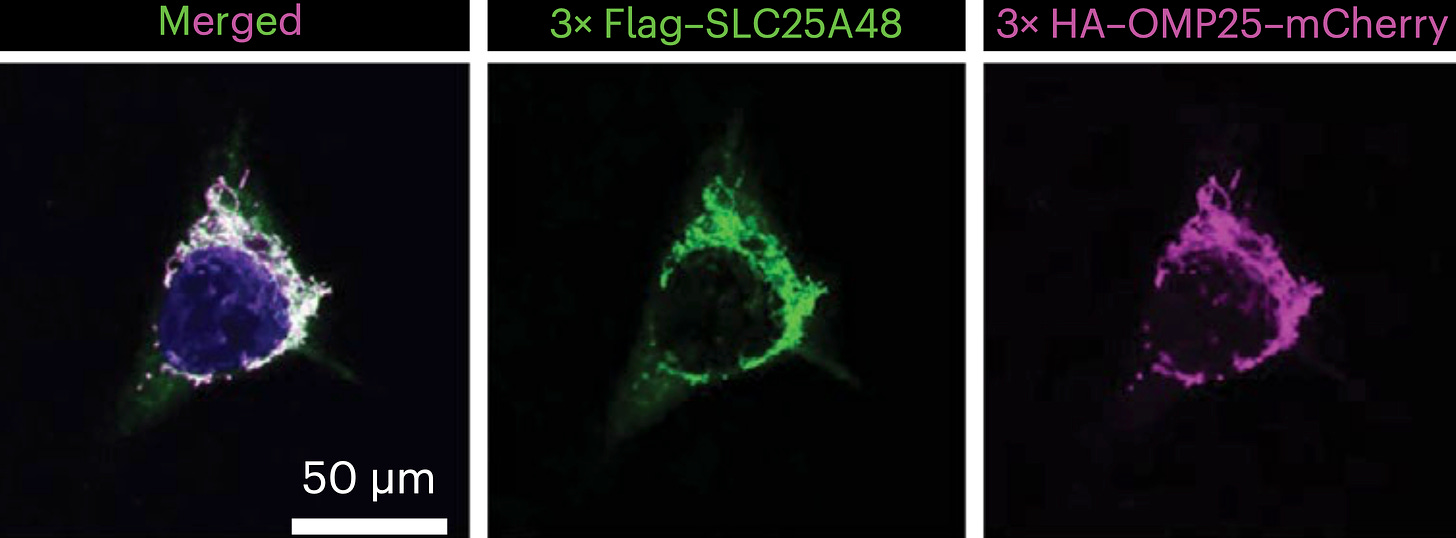
The human genome has around 18,000 to 20,000 genes that code for proteins. I often wonder how much the field has learned about the function(s) of each of these genes. One thing about human genetics that has never ceased to amaze me is how naturally occurring spelling errors in the gene sequences in the population shed light on the normal functions of the genes. The value of something is appreciated only when it is lost. Many of the genes in the human genome were known to exist today only because scientists encountered humans in the past in whom such genes didn't exist, functionally speaking.
One class of genes that I find particularly interesting are the ones that encode transporters and ion channels. Millions of molecules are on a constant move in the human body, and often their movements are facilitated by transporters and channel proteins. They let molecules shuttle in and out of cells and cell organelles. Sometimes the cells merely act as passages through which molecules enter into or exist out of the human body to conduct their day-to-day businesses. Genetic mutations that partially or completely block such passages create traffic jams, leading to crowding of the passengers either inside or outside the body and send signals to scientists, setting the stage for new discoveries.
One channel protein that probably comes to everyone's mind with the mention of human genetics is cystic fibrosis gene CFTR. It encodes chloride channels in the cells lining the walls of the ducts that allow air to flow into the lungs, digestive enzymes to flow through the pancreas and sweat to be released from sweat glands. Loss of this channel blocks the movement of chloride and sodium and consequently, water, across the ductal epithelial cells, dehydrating and driving up the viscosity of the ductal contents.
One of the key pieces of the cystic fibrosis puzzle that helped scientists triangulate the genetic cause appeared during a hot summer in 1948 when a heat wave swept through the New York. Paul di Sant'Agnese, a pediatrician at the New York-Presbyterian Hospital, noticed that cystic fibrosis patients were disproportionately exhausted by the heat than other patients in the hospital. This observation led to the discovery of excessive loss of salt (sodium and chloride) in the sweat in cystic fibrosis patients, and subsequent realization that the core problem in cystic fibrosis might be related to ion channels.
The fact that the sweat glands do not retain salt effectively in hot weather, during salt restriction or when DCA [deoxycorticosterone acetate] is administered, points to a disturbance in the sweat glands themselves. This abnormality may not be pathognomonic of cystic fibrosis of the pancreas, but it is an expected finding.
These disturbances in sweat composition together with preliminary observations of abnormal salivary gland secretion suggest that the secretory activity of many and perhaps all exocrine glands is affected in cystic fibrosis of the pancreas. The term "mucoviscidosis" does not seem justified in view of the fact that at least two nonmucus-secreting structures are affected in this condition
One of the examples that first come to mind when I think of human genetics and transporters is the discovery of uric acid transporters. Uric acid is an end product of purine metabolism (breakdown of adenine and guanine nucleotide bases). Uric acid is primarily excreted through kidneys. The blood levels of uric acid are determined by the balance between its rates of production and excretion. Abnormally high levels of uric acid in the blood is bad. It leads to deposition of uric acid crystals in the tissues and joints, resulting in gouty arthritis.
The knowledge of gout itself is remarkably ancient, known to exist for thousands of years. The link between uric acid and gout is also known for nearly two centuries now. However, the knowledge of key transporters in the kidneys that titrate the uric acid levels in the blood remained a mystery until the early 2000s. Then the human genome project happened, laying the map of human genome for scientists to navigate through in pursuit of new genes.
The uric acid that escape through the glomerulus of the nephrons in the kidney gets reabsorbed back into the system through the cells lining the tubular portions of the nephrons. This process is accomplished by two transporters: one embedded on the side facing the inside of the tubes (apical surface) and the other facing the blood circulation (basolateral surface).
The transporter on the apical surface, SLC22A12, was discovered in 2002 through a systematic search across the genome for transporter-like gene sequences, followed by molecular characterization and animal experiments (Enomoto et al. Nature 2002). Only after learning the apical surface location of SLC22A12, scientists realized that there should be another similar transporter on the basolateral side that shuttles the uric acid into the blood circulation (shown as a question mark in the illustration below).
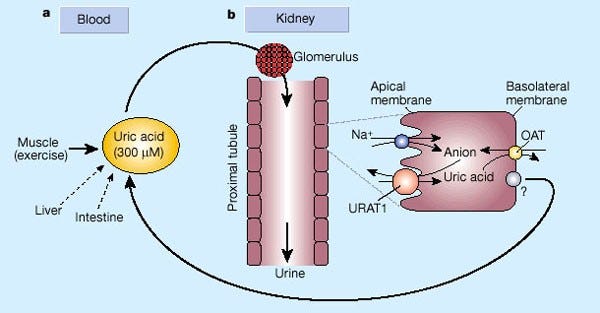
In 2008, three genome-wide association studies (GWAS) in parallel reported the discovery of a strong genetic association between SLC2A9, encoding a presumed glucose transporter GLUT9, and serum uric acid levels (Wallace et al. AJHG 2008; Vitart et al. Nat Gen 2008; Döring et al. Nat Gen 2008). It turned out, GLUT9 was primarily a urate transporter, the mystery protein sitting on the basolateral side of the renal tubules and working in partnership with SLC22A12 to reabsorb uric acid from the glomerular filtrate.
Today, we know there are a bunch of urate transporters sitting on the renal tubular cells and genetic variations near genes encoding almost all of these proteins have a striking influence on the uric acid levels in the blood in humans, which you can beautifully see as skyscrapers in a GWAS Manhattan plot. Even if molecular biologists did not discover these transporters early on, they would have eventually come to light through GWAS.
There are hundreds of metabolites like uric acid in the blood, and have we figured out all the transporter genes shuttling these metabolites across various sites in the human body? A recent discovery reminds us the answer to that question.
See, discoveries of the chloride channel and urate transporters were heavily inspired by the associated diseases. The discoveries happened as scientists were aggressively studying the root causes of the cystic fibrosis and gout. There are probably hundreds of transporters whose roles have not yet been realized because disruption of their functions either do not lead to major disease states or the associated diseases have not yet been linked to the causal metabolites. And all it takes is a small hint, for example, as in the recent discovery where scientists picked up on a genetic signal linked to plasma and urine choline levels and ended up discovering the human mitochondrial choline transporter.
In the past few months, at least three research groups have independently discovered that there exists a transporter protein, encoded by SLC25A48, in the mitochondrial membrane that is required for choline to get inside the mitochondria to do its business (Patil et al. medRxiv 2024; Khan et al. Nat Gen 2008; Verkerke et al. bioRxiv 2024). When this transporter doesn't work, choline levels spike up in the blood and urine.
Choline is an essential nutrient, a vitamin-like chemical required for many important biological processes such as synthesis of phosphatidylcholine (a component of cell membrane), synthesis of neurotransmitters (e.g. acetylcholine) and one carbon metabolism (e.g. folate metabolism).
The fascinating part of the discovery of choline transporter is that scientists weren’t even looking for them. Probably, no one even realized that there existed another transporter for choline (a few choline transporters were already known to exist). That's the beauty of hypothesis-free studies such as GWAS. You go in there blind and stumble upon something new, pointing to an important biology.

One of the three research groups that discovered the choline transporter is from the University of Freiburg in Germany, who conducted GWASs of 1,916 plasma and urine metabolites in 2023 (Schlosser et al. Nat Gen 2023). One of the 1,299 genetic signals they identified was found near an orphan transporter protein SLC25A48, associated with urine choline levels. They followed up this finding further and found that the genetic variants near SLC25A48 are not only mQTLs for choline in the urine, they are also mQTLs for choline in the plasma and eQTLs for SCL25A48 in the brain tissue. They decided to dive deep into the signal and performed various functional experiments and arrived at the conclusion that SLC25A48 is a choline transporter located in the inner mitochondrial membrane, and a major regulator of plasma choline levels.
The second group is from the Rockefeller and Vanderbilt Universities in the US, who also spotted the SLC25A48 signal through GWAS of plasma metabolome. The authors first grouped the significant associations into two groups: ones for which the causal genes are clear based on prior literature and the ones for which causal genes aren't clear, hence provide opportunities to uncover new biology. They decided to study the top most signal in the second pile: SLC25A48 associated with plasma choline. Then followed a series of in vitro experiments, which led to the revelation that SLC25A48 is a mitochondrial choline transporter. The authors also report possible health consequences of SLC25A48 dysfunction by performing a phenome-wide association study of rare loss of function variants and common variants near SLC25A48. The associations include diseases of nervous system, bone, tooth, skin and blood vessels.
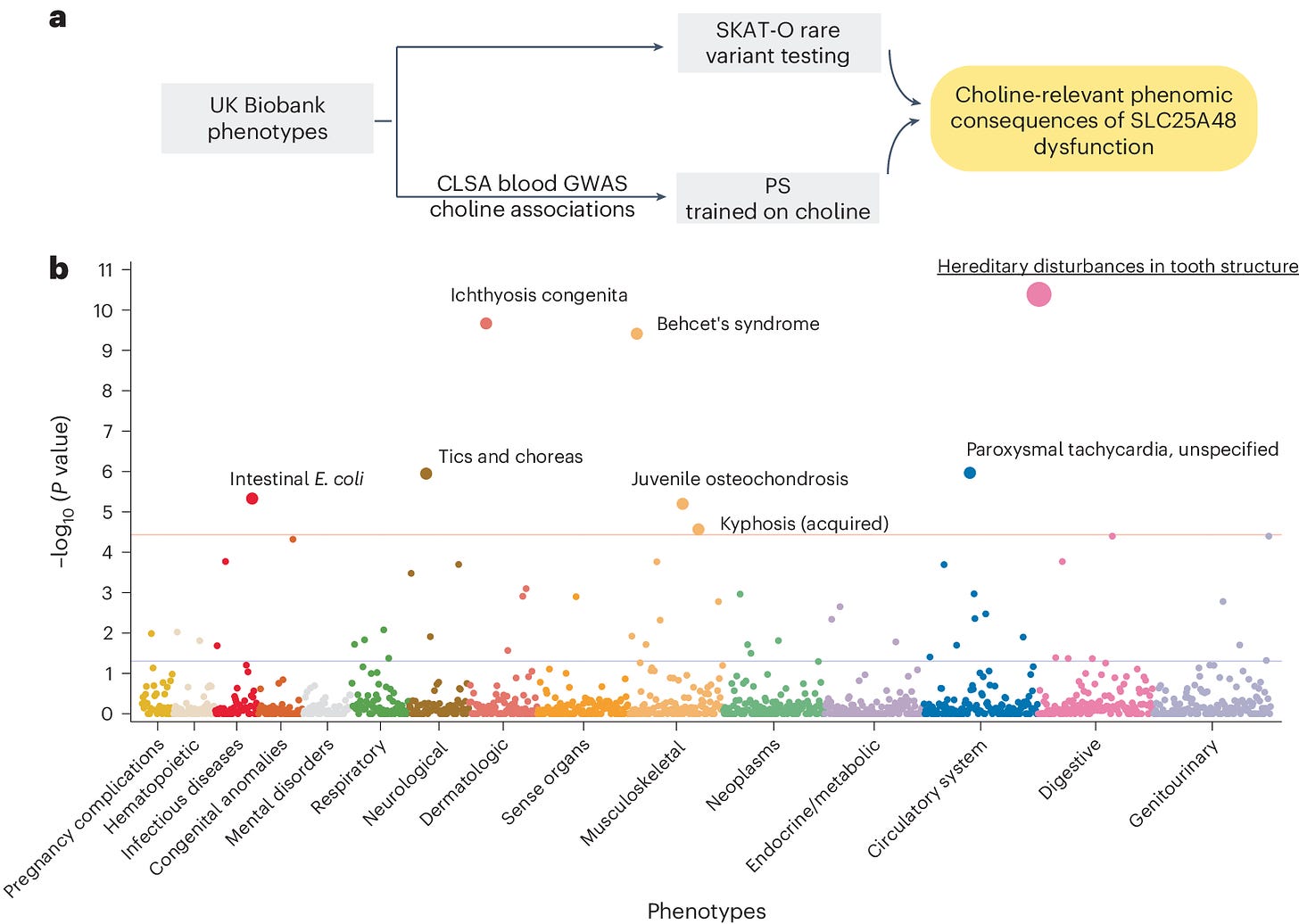
The third group is from Beth Israel Deaconess Medical Center and Harvard Medical School in the US, who landed on SLC25A48 without the help from human genetics. The authors performed a proteomics analysis of mouse brown adipose tissue in the context of diet-induced obesity and ended up discovering SLC25A48, which was significantly upregulated in the brown adipose tissue in a high-fat diet. Long story short, the authors studied the mitochondrial respiratory function in Slc25a48 knock out mice and somehow discovered the link to choline and arrived at the same conclusion as other two groups.
It is fascinating to see a biological finding remains hidden for a long time and then suddenly it comes to light with a bang. Studies like these are reminders that there is still a lot more exciting biology remain unknown to the world, hidden beneath massive amounts of genomic data, waiting to be discovered.